Emeritus Professor Suresh Bhatia
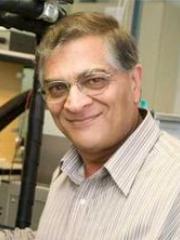
Researcher biography
Biography:
Suresh Bhatia received a B.Tech. degree in Chemical Engineering from the Indian Institute of Technology, Kanpur, and Master's as well as PhD degrees from the University of Pennsylvania. He worked for a few years in industry in the USA, and for two years at the University of Florida, before joining the Indian Institute of Technology, Mumbai, in 1984, and subsequently The University of Queensland in 1996. His main research interests are in adsorption and transport in nanoporous materials and in heterogeneous reaction engineering, where he has authored over two hundred and eighty scientific papers in leading international journals. He has received numerous awards for his research, including the Shanti Swarup Bhatnagar Prize for Engineering Sciences from the Government of India, and the ExxonMobil Award for excellence from the Institution of Chemical EngineersHe has held an Australian Professorial Fellowship from the Australian Research Council, and is a Fellow of two major academies – the Australian Academy of Technological Sciences and Engineering, and the Indian Academy of Sciences. He served as the Regional Editor of the international journal Molecular Simulation between 2009 and 2015. He has held visiting positions at leading universities, and between 2007 and 2009 he was the Head of the Division of Chemical Engineering at UQ.
Research:
Bhatia's main research interests are in the modelling and simulation of adsorption and transport in nanoporous materials, and in heterogeneous reaction engineering, in which he pursues both applied and fundamental research on a variety of topics. One of the current subjects is the development of models for the reaction kinetics and transport processes in the green electrocatalytic reduction of carbon dioxide, as part of the research activities of the Australian Research Council Centre of Excellence. This is a novel route to reducing carbon dioxide emissions by converting it to useful chemicals and fuels, that is rapidly gaining increasing interest. In this technique, a porous electrode of complex structure is coated with nanoparticles of an electrocatalyst, on the surface of which carbon dioxide is reduced. Carbon dioxide (either pure gas or as part of flue gas) is fed into the electrolyser and must diffuse through the electrode's structure to react with hydrogen ions in a liquid-phase electrolyte at the surface of the electrocatalyst. An added complexity is the intrusion of the electrolyte into the electrode, leading to its flooding and to a reduction in gas-phase transport rates. Bhatia's research aims to gain an understanding of the complex interplay between gas-phase and electrolyte transport, and interfacial reaction kinetics, combining nanoscale models of transport and electrocatalytic kinetics with macroscopic electrode level models, and develop a comprehensive approach useful for process design and scale-up.
A second stream of activity relates to the modelling of mixed matrix membranes, particularly for carbon dioxide separation from flue gas and other industrial gas streams. These are a new class of membranes comprising a nanoporous adsorbent filler such as a zeolite or metal-organic/zeolitic imidazole framework material dispersed in a polymer matrix. Such composite membranes combine the high flux capabilities of the adsorbent with the selective properties of the polymer to overcome the established Robeson upper bound for polymers. Bhatia has developed novel effective medium theory-based models for transport in finite-sized composites, which overcome limitations of existing theories that are applicable only to large systems and therefore overlook particle and system size effects. At a more molecular level, Bhatia is investigating the nanoscale interfacial structure of the polymer in the vicinity of the solid, and its influence on the interfacial transport resistance using molecular dynamics simulation methods. When the polymer-filler interaction is strong, there is local densification of the polymer, which hinders gas transport, and when this interaction is weak interfacial nano-voids are formed which reduces selectivity. Both of these distinct effects deteriorate membrane performance, and a current focus of our research is the functionalisation of the polymer to improve polymer-filler compatibility and reduce interfacial defects. The synthesis of nanoscale and macroscopic approaches holds promise for the development of a virtual tool for the De Novo design of mixed matrix membrane specific to a given separation application; and is a key goal of this research.
Another thrust of his research relates to the transport of fluids in nanopores and nanoporous materials, where he is developing practical models of transport in nanoporous materials in conjunction with simulation and experiment. Among the achievements is a new theory of transport in nanoscale pores, which leads to an exact new result at low densities superseding the century-old Knudsen model. A current focus of the research is the interfacial resistance to transport in nanpororous materials, using molecular dynamics simulations and theoretical techniques. His results have shown that interfacial resistance dominates at nanoscales and can be very significant even at microscales. The results will have importance for a range of nanotechnologies involving the infiltration of fluids in nanoporous materials, including catalysis, gas storage, adsorption, and membrane-based separations, as well as nanofluidics.
In another stream of activity, he has developed atomistic models of disordered carbons using hybrid reverse Monte Carlo simulation methods, in conjunction with neutron scattering experiments. These atomistic models have been used to investigate the adsorption and transport of adsorbed fluids in the carbon nanostructure for a variety of applications. Among the carbons examined are carbide-derived carbon-based adsorbents for carbon dioxide capture from moist flue gases and CH4/CO2 separations. The co-adsorption of water has been shown by him to have a critical influence on both equilibrium and transport properties in these applications, and strategies for mitigating this influence are being investigated by means of simulation.
An area of recent activity is the study of carbon supercapacitors, where he is developing advanced simulation-based models for the equilibrium and flow of ions in porous carbon electrodes. These models will enable the optimisation of carbon structure for maximising capacitance and enhancing charging/discharging rates.
Teaching and Learning:
Bhatia has teaching interests in chemical reaction engineering, and applied mathematics, both at the undergraduate and postgraduate levels.
Projects:
- Simulation of the kinetics of electrocatalytic reduction of carbon dioxide. The electrocatalytic transformation of carbon dioxide to useful chemicals and fuels is a subject of much current interest to the goal of a net zero carbon economy. This project aims to develop a model of the kinetics of the electrocatalytic reaction and use it to optimise the structure and loading of the electrocatalyst layer on the surface of the electrode. A combination of Quantum calculations and kinetic Monte Carlo simulations will be performed to determine the reaction kinetics for the carbon dioxide reduction to specific products such as ethylene and urea. Machine learning will be used to correlate intrinsic reaction kinetics with ionic concentrations in the electrolyte. Subsequently, reaction-diffusion modelling in the electrolyte will be performed to determine the optimal properties of the catalyst layer for maximising production rates. Validation of the models will be conducted using experimental data from other groups in the ARC Centre of Excellence for Green Carbon Dioxide Transformation.
- Multiphase transport in packings of nanospheres. Numerous materials comprise packings of nano-sized particles. Examples are catalytically active layers of metals deposited on surfaces, layers of carbon nanoparticles in electrodes, and extrudates of catalyst and adsorbent particles comprised of aggregated nanoparticles. Current models of transport through such materials often simplify the structure by appealing to an idealised cylindrical pore model, which is often inaccurate and requires the use of empirical fitting parameters. In addition, such models frequently overlook fluid-solid interactions that become important at nanoscales. This project will investigate simultaneous gas and liquid electrolyte transport in packings of nanospheres, while considering fluid-solid interaction and phase equilibrium between gas and liquid, using molecular dynamics simulations, to determine multiphase transport properties as a function of interaction parameters, packing structure, packing density and particle size, and the results corelated using machine learning models. The models developed will be useful in the design of catalyst and adsorbent particles, and of electrodes in electrochemical processes.
- Modelling transport in diffusion electrodes. Numerous electrochemical systems, such as fuel cells and electrocatalytic reactors use electrodes of complex structure, comprising a fibrous gas diffusion layer, a conductive carbon particle layer and a catalytic layer. The electrode separates gas and liquid electrolyte, both of which infiltrate the electrode from opposite sides. A reliable model of the electrode behaviour is essential for process design. This project will model the interplay between gas and electrolyte transport and their phase equilibrium in the electrode, as well as the reaction-diffusion process in the catalytic layer facilitated by the charge transport in the electrode. Joule heating of the electrode will also be considered. The particular process targeted is the electrocatalytic conversion of carbon dioxide. The outcome will be a comprehensive model of reaction and transport in the electrode that can be used in process design and scale-up of the electrochemical cell for electrocatalytic carbon dioxide reduction.
- Synthesis and modelling of mixed matrix membranes. Mixed matrix membranes comprising a zeolite, metal-organic framework material, or other suitable adsorbent dispersed within a polymer matrix are attracting considerable attention because they combine the good mechanical properties of the polymer matrix with separation properties of the adsorbent. Here, we will perform molecular dynamics simulations of the separation of CO2 from flue gas using mixed matrix membranes and investigate their transport properties in this application. Suitable functionalisation of the polymer will be performed in silico to alleviate interfacial defects. Machine learning will be used to correlate transport properties with fundamental molecular level polymer and filler properties. Mathematical models of permeation through the membrane will be developed and validated against experimental data.
- Dynamics of mixture adsorption in nanoporous materials. This project focuses on understanding the diffusion of gases in nanoporous materials, which is challenging both from a fundamental and applications viewpoint. Existing models frequently overlook fluid-solid interactions and require fitting parameters. In this connection, we have already performed molecular dynamics studies with single component systems and developed a novel new theory of diffusion and transport of adsorbates in nanoporous materials. The new studies now proposed focus on gas mixtures, and the theory developed will be extended to multicomponent systems in conjunction with molecular dynamics simulation. A system of particular interest is the separation of carbon dioxide from flue gas using nanomaterials and membranes.